Transposon: Difference between revisions
imported>David Tribe |
imported>Caesar Schinas m (Bot: Delinking years) |
||
(40 intermediate revisions by 5 users not shown) | |||
Line 1: | Line 1: | ||
{{subpages}} | |||
[[Image:Maizemutatorsmall.jpg|right|frame|Heavy spotting on corn kernels reveals the activity of the Mutator transposon system. Photo: Damon Lisch PLoS Biology Open Access License <ref> [http://biology.plosjournals.org/perlserv/?request=get-document&doi=10.1371/journal.pbio.0040353 Transposon Silencing Keeps Jumping Genes in Their Place Gross L PLoS Biology Vol. 4, No. 10, e353 doi:10.1371/journal.pbio.0040353]</ref>.]] | |||
'''Transposons''', also called "jumping genes", "[[mobile DNA]]" or "mobile [[genetics|genetic]] elements", are blocks of conserved [[DNA]] that can occasionally move to different positions within the [[chromosome]]s of a [[cell (biology)|cell]]. The insertion of a transposon in a new site is called '''transposition'''. It can result in DNA duplication and in further multiplication of the original DNA sequence. The transposition process can cause [[mutation]]s at the site of DNA insertion, and can cause increases in the total amount of DNA in a genome. Transposons were first discovered by [[Barbara McClintock]] in 1942-1948 <ref>[http://profiles.nlm.nih.gov/LL/ The Barbara McClintock Papers, National Library of Medicine: McClintock, B. "Cytogenetic Studies of Maize and Neurospora." Carnegie Institution of Washington Yearbook 44, (1945): 108-112. McClintock, B. "Maize Genetics." Carnegie Institution of Washington Yearbook 45, (1946): 176-186. McClintock, B. "Mutable Loci in Maize." Carnegie Institution of Washington Yearbook 47, (1948): 155-169.]</ref>, and she was eventually awarded the [[Nobel Prize]] in 1983 for this discovery <ref>[http://newton.nap.edu/html/biomems/bmcclintock.html BIOGRAPHICAL MEMOIRS National Academy of Sciences: Barbara McClintock June 16, 1902 — September 2, 1992 By Nina V. Fedoroff]</ref>. | |||
Transposons | Transposons insert at new locations in DNA by enzymatic breakage and reunion of DNA, a process called DNA [[recombination]]. The distinctive feature of all transposon movement is that there is no requirement for extensive DNA sequence similarity between the initial DNA region containing the transposon and the final location (that is to say, extensive [[base pair]] complementarity), and the process of transposon insertion at a new location is considered to be non-homologous [[recombination]] to distinguish it from [[recombination]] of similar DNA sequences participating in homologous [[recombination]]. | ||
Transposons make up a large fraction of [[genome | There are a variety of mobile genetic elements, and they can be broadly classified by their mechanism of transposition. Class I mobile genetic elements, or [[retrotransposon]]s, move in the genome by being '''[[Transcription (genetics)|transcribed]] to [[RNA]]''' and then transcribed back to DNA catalyzed by the enzyme [[reverse transcriptase]], while class II mobile genetic elements move directly from one position to another within the genome using a [[transposase]] enzyme catalyst to mobilize the DNA ''without involving an RNA intermediate''. | ||
For researchers, transposons are a very useful method to alter DNA inside of a living organism. | |||
Transposons make up a large fraction of the total [[genome]] of many animals and plants which is evident through the [[C-value]]s of [[eukaryote|eukaryotic]] species. As an example, about 45% of the [[human genome]] is composed of transposons and their defunct remnants <ref>[http://www.nature.com/nature/journal/v409/n6822/pdf/409860a0.pdf International Human Genome Sequencing Consortium Initial sequencing and analysis of the human genome NATURE VOL 409 15 FEBRUARY 2001, p 860-921] </ref>. | |||
==Types of transposons== | ==Types of transposons== | ||
Line 14: | Line 18: | ||
===Class I: Retrotransposons=== | ===Class I: Retrotransposons=== | ||
{{main|Retrotransposon}} | {{main|Retrotransposon}} | ||
Retrotransposons work by [[copy and paste|copying themselves and pasting copies]] back into the genome in multiple places. Initially retrotransposons copy themselves to [[RNA]] ([[Transcription (genetics)|transcription]]) but, in addition to being translated, the RNA is copied into DNA by a [[reverse transcriptase]] (often coded by the transposon itself) and inserted back into the genome. | Retrotransposons work by [[copy and paste|copying themselves and pasting copies]] back into the genome in multiple places. Initially, retrotransposons copy themselves to [[RNA]] ([[Transcription (genetics)|transcription]]) but, in addition to being translated, the RNA is copied into DNA by a [[reverse transcriptase]] (often coded by the transposon itself) and inserted back into the genome. | ||
Retrotransposons behave very similarly to retroviruses, such as [[HIV]], giving a clue to the [[evolution]]ary origins of such viruses. Retrotransposons are common in eukaryotic | Retrotransposons behave very similarly to retroviruses, such as [[HIV]], giving a clue to the [[evolution]]ary origins of such viruses. Retrotransposons are common in eukaryotic organism s(for instance maize, humans), but are rarely found in bacteria. They are present in fungi. | ||
There are four main classes of | There are four main classes of [[retrotransposon]]s: | ||
*Viral superfamily: similar to retroviruses, have long terminal repeats (LTRs), encode reverse transcriptase (to reverse transcribe RNA into DNA). | *Viral superfamily: similar to retroviruses, have long terminal repeats (LTRs), encode reverse transcriptase (to reverse transcribe RNA into DNA). | ||
*LINES: encode reverse transcriptase (to reverse transcribe RNA into DNA), lack LTRs, transcribed by [[RNA polymerase II]]. | *LINES: encode reverse transcriptase (to reverse transcribe RNA into DNA), lack LTRs, transcribed by [[RNA polymerase II]]. | ||
*SINES: short elements that do encode reverse transcriptase but are otherwise similar to LINEs. Include the human Alu elements. | *SINES: short elements that do encode reverse transcriptase but are otherwise similar to LINEs. Include the human Alu elements. | ||
*Nonviral superfamily: do not code for reverse transcriptase, transcribed by [[RNA polymerase III]] | *Nonviral superfamily: do not code for reverse transcriptase, transcribed by [[RNA polymerase III]]. | ||
===Class II:DNA | ===Class II:DNA transposons=== | ||
The major difference of Class II transposons from retrotransposons is that their transposition mechanism does not involve an RNA intermediate. Class II transposons usually move by [[cut and paste]], rather than copy and paste, using the [[transposase]] enzyme. Different types of transposase work in different ways. Some can bind to any part of the DNA molecule, and the target site can therefore be anywhere, while others bind to specific sequences. Transposase makes a staggered cut at the target site producing sticky ends, cuts out the transposon and ligates it into the target site. A [[DNA polymerase]] fills in the resulting gaps from the sticky ends and [[DNA ligase]] closes the sugar-phosphate backbone. This results in target site duplication and the insertion sites of DNA transposons may be identified by '''short direct repeats''' (a staggered cut in the target DNA filled by DNA polymerase) which flank the '''inverted repeats''' that are part of the transposon itself (and which are important for the transposon excision by transposase). | The major difference of Class II transposons from retrotransposons is that their transposition mechanism does not involve an RNA intermediate. Class II transposons usually move by [[cut and paste]], rather than copy and paste, using the [[transposase]] enzyme. Different types of transposase work in different ways. Some can bind to any part of the DNA molecule, and the target site can therefore be anywhere, while others bind to specific sequences. Transposase makes a staggered cut at the target site producing sticky ends, cuts out the transposon and ligates it into the target site. A [[DNA polymerase]] fills in the resulting gaps from the sticky ends and [[DNA ligase]] closes the sugar-phosphate backbone. This results in target site duplication and the insertion sites of DNA transposons may be identified by '''short direct repeats''' (a staggered cut in the target DNA filled by DNA polymerase) which flank the '''inverted repeats''' that are part of the transposon itself (and which are important for the transposon excision by transposase). | ||
Not all DNA transposons transpose through cut and paste mechanism. In some cases | Not all DNA transposons transpose through cut and paste mechanism. In some cases [[replicative transposition]] is observed in which transposon replicates itself to a new target site without being excised from its original site. In this process a [[cointegrate]] structure is formed containing the donor and target elements covalently connected to one-another, and in this replicative transposition resembles homologous recombination. Examples of transposons that use replicative transposition include bacteriophage Mu, Tn3 and IS1. | ||
Both class I and class II of transposons may lose their ability to | Both class I and class II of transposons may lose their ability to synthesize reverse transcriptase or transposase through mutation, yet continue to jump through the genome because other transposons are still producing the necessary enzyme. Transposons that themselves encode an ability to move to new locations are called '''autonomous''' transposons. | ||
=== Class III: Miniature | === Class III: Miniature inverted-repeat transposable elements === | ||
'''MITEs''' are sequences of about 400 base pairs and 15 base pair inverted repeats that vary very little. They are found in their thousands in the genomes of both [[plants]] and [[animals]] (over 100,000 were found in the [[rice]] genome). MITEs are too small to encode any [[protein]]s. | '''MITEs''' are sequences of about 400 base pairs and 15 base pair inverted repeats that vary very little. They are found in their thousands in the genomes of both [[plants]] and [[animals]] (over 100,000 were found in the [[rice]] genome). MITEs are too small to encode any [[protein]]s. | ||
== Examples == | == Examples == | ||
* The first transposons were discovered in [[maize]] (''Zea mays''), (aka corn) by [[Barbara McClintock]] in the 1940s, for which she was awarded | * The first transposons were discovered in [[maize]] (''Zea mays''), (aka corn) by [[Barbara McClintock]] in the 1940s, for which she was awarded the [[Nobel Prize]] in 1983. She noticed the results of [[insertion (genetics)|insertion]]s, [[deletion]]s, and [[translocation]]s, caused by these transposons. These changes in the genome could, for example, lead to a change in the color of corn kernels. About 50% of the total genome of maize consists of transposons. The Ac/Ds system McClintock described are class II transposons <ref>Bennetzen, J. L., 2000 Transposable element contributions to plant gene and genome evolution. Plant Molecular Biology 42: 251–269, 2000.</ref>. | ||
* One family of transposons in the fruit fly ''[[Drosophila melanogaster]]'' are called ''[[P element]]s''. They seem to have first appeared in the [[species]] only in the middle of the twentieth century. Within 50 years, they have spread through every [[population]] of the species. Artificial P elements can be used to insert genes into Drosophila by injecting the [[embryo]]. For the use of P elements as a genetic tool see: "[[transposons as a genetic tool]]". | * One family of transposons in the fruit fly ''[[Drosophila melanogaster]]'' are called ''[[P element]]s''. They seem to have first appeared in the [[species]] only in the middle of the twentieth century. Within 50 years, they have spread through every [[population]] of the species. Artificial P elements can be used to insert genes into Drosophila by injecting the [[embryo]]. For the use of P elements as a genetic tool see: "[[transposons as a genetic tool]]". | ||
* The simplest form of bacterial transposon are [[insertion sequence]]s (IS) which are about 1000 base pairs long and consist of inverted DNA repeats flanking a transposase gene. Other transposons in [[bacterium|bacteria]] usually carry an additional gene for function other than transposition---often for [[antibiotic resistance]]. They can consist of two IS elements flanking a gene for antibiotic resistance <ref>Snyder, L. and Champness, W. (2003) | * The simplest form of bacterial transposon are [[insertion sequence]]s (IS) which are about 1000 base pairs long and consist largely of inverted DNA repeats flanking a transposase gene. Other more complex transposons in [[bacterium|bacteria]] usually carry an additional gene for function other than transposition---often for [[antibiotic resistance]]. They can consist of two IS elements flanking a gene for antibiotic resistance <ref>Snyder, L. and Champness, W. (2003)Transposon and site-specific recombination, Chapter 9 In Snyder, L. and Champness, W. ''Molecular Genetics of Bacteria''. 2nd. Edition. ASM Press, Washington, DC.</ref>. IS elements were first discovered in the ''gal'' gene of ''Escherichia coli'' by James Shapiro in 1968 <ref> Shapiro, J.A. (1968). Mutations caused by insertion of genetic material into the galactose operon of ''Escherichia coli''. Journal of Molecular Biology 40, p93-105.</ref>. | ||
* Stimulated by Shapiro's 1968 report, follow-up [[molecular biology]] research on transposon involvement in numerous mobile DNA related | * Stimulated by Shapiro's 1968 report, follow-up [[molecular biology]] research on transposon involvement in numerous mobile DNA related phenomena in bacteria, plants and insects<ref>Berg, D. E. and Howe, M. M. ''Mobile DNA'' ASM Press, Washington DC.</ref> finally led to Barbara McClintock's earlier discoveries with maize being given wide acclaim among biologists. | ||
* In bacteria, transposons can jump from [[chromosome|chromosomal]] DNA to [[plasmid]] DNA and back, allowing for the transfer and permanent addition of genes such as those encoding antibiotic resistance ([[multidrug resistance|multi-antibiotic resistant]] bacterial strains can be generated in this way). Bacterial transposons of this type belong to the Tn family. | * In bacteria, transposons can jump from [[chromosome|chromosomal]] DNA to [[plasmid]] DNA and back, allowing for the transfer and permanent addition of genes such as those encoding antibiotic resistance ([[multidrug resistance|multi-antibiotic resistant]] bacterial strains can be generated in this way). Bacterial transposons of this type belong to the Tn family. | ||
* Mu phage in the bacterium ''Escherichia coli'' is a well known example of [[replicative transposition]]. It can exist as a [[prophage]] inserted like a transposon in the ''E. coli'' genome. Its replication by transposition mechanism leaves a copy of the prophage at the | * Mu [[phage]] in the bacterium ''Escherichia coli'' is a well known example of [[replicative transposition]]. It can exist as a [[prophage]] inserted like a transposon in the ''E. coli'' genome. Its replication by transposition mechanism leaves a copy of the prophage at the original location during transposition.<ref> Ljungquist, E. and Bukhati, A. I.(1977) State of prophage Mu upon induction. PNAS USA 74, p3143-3147).</ref> | ||
* The most common form of transposon in [[human]]s is the [[Alu sequence]] a form of SINE. The [[Alu sequence]] is approximately 300 bases long and can be found | * The most common form of transposon in [[human]]s is the [[Alu sequence]], a form of SINE. The [[Alu sequence]] is approximately 300 bases long and about 1000 copies of Alu can be found in the human [[genome]] <ref>[http://www.nature.com/nature/journal/v409/n6822/pdf/409860a0.pdf International Human Genome Sequencing Consortium Initial sequencing and analysis of the human genome NATURE VOL 409 15 FEBRUARY 2001, p 860-921] </ref>. | ||
== Transposons causing diseases == | == Transposons causing diseases == | ||
Line 54: | Line 58: | ||
* A transposon or a retroposon that inserts itself into a functional gene will most likely disable that gene. | * A transposon or a retroposon that inserts itself into a functional gene will most likely disable that gene. | ||
* After a transposon leaves a gene, the resulting gap will probably not be repaired correctly. | * After a transposon leaves a gene, the resulting gap will probably not be repaired correctly. | ||
* Multiple copies of the same sequence, such as [[Alu sequence]]s can hinder precise [[chromosome|chromosomal]] pairing during [[mitosis]], resulting in unequal [[chromosomal crossover|crossover]]s, one of the main reasons for chromosome duplication. | * Multiple copies of the same sequence, such as [[Alu sequence]]s can hinder precise [[chromosome|chromosomal]] pairing during [[mitosis]], resulting in unequal [[chromosomal crossover|crossover]]s, one of the main reasons for chromosome duplication <ref>Abeysinghe SS, Chuzhanova N, Krawczak M, Ball EV, Cooper DN.(2003) Translocation and gross deletion breakpoints in human inherited disease and cancer I: Nucleotide composition and recombination-associated motifs. Hum Mutat. 2003 Sep;22(3):229-44.</ref> <ref>Kolomietz E, Meyn MS, Pandita A, Squire JA. (2002) The role of Alu repeat clusters as mediators of recurrent chromosomal aberrations in tumors.Genes Chromosomes Cancer. 2002 Oct;35(2):97-112.</ref>. | ||
Diseases that are often caused by transposons include [[hemophilia]] A and B, [[severe combined immunodeficiency]], [[porphyria]], predisposition to [[cancer]], and [[Duchenne muscular dystrophy]]. | Diseases that are often caused by transposons include [[hemophilia]] A <ref>Ganguly A, Dunbar T, Chen P, Godmilow L, Ganguly T. (2003) Exon skipping caused by an intronic insertion of a young Alu Yb9 element leads to severe hemophilia A. Hum Genet. 2003 Sep;113(4):348-52. Epub 2003 Jul 12. PMID: 12884004 </ref> and B <ref>Li X, Scaringe WA, Hill KA, Roberts S, Mengos A, Careri D, Pinto MT, Kasper CK, Sommer SS.(2001) Frequency of recent retrotransposition events in the human factor IX gene.Hum Mutat. 2001 Jun;17(6):511-9. </ref>, [[severe combined immunodeficiency]]<ref>Markert ML, Hutton JJ, Wiginton DA, States JC, Kaufman RE. (1988) Adenosine deaminase (ADA) deficiency due to deletion of the ADA gene promoter | ||
and first exon by homologous recombination between two Alu elements. J Clin Invest. 1988 May;81(5):1323-7.</ref>, [[porphyria]]<ref>Mustajoki S, Ahola H, Mustajoki P, Kauppinen R.(1999) Insertion of Alu element responsible for acute intermittent porphyria.Hum Mutat. 1999;13(6):431-8.PMID: 10408772</ref>, predisposition to [[cancer]]<ref>Teugels E, De Brakeleer S, Goelen G, Lissens W, Sermijn E, De Greve J.(2005) De novo Alu element insertions targeted to a sequence common to the BRCA1 and BRCA2 genes.Hum Mutat. 2005 Sep;26(3):284.</ref> <ref>Gibbons R, Dugaiczyk A.(2005) Phylogenetic roots of Alu-mediated rearrangements leading to cancer. Genome. 2005 Feb;48(1):160-7.</ref>, and possibly [[Duchenne muscular dystrophy]]<ref>McNaughton JC, Hughes G, Jones WA, Stockwell PA, Klamut HJ, Petersen GB.(1997) The evolution of an intron: analysis of a long, deletion-prone intron in the | |||
human dystrophin gene.Genomics. 1997 Mar 1;40(2):294-304.</ref>. | |||
Additionally, many transposons contain promoters which drive [[Transcription (genetics)|transcription]] of their own [[transposase]]. These promoters can cause aberrant expression of linked genes, causing disease or [[mutant]] [[phenotypes]]. | Additionally, many transposons contain promoters which drive [[Transcription (genetics)|transcription]] of their own [[transposase]]. These promoters can cause aberrant expression of linked genes, causing disease or [[mutant]] [[phenotypes]]. | ||
== Evolution of transposons == | == Evolution of transposons == | ||
The evolution of transposons and their effect on genome evolution is currently a | The evolution of transposons and their effect on genome evolution is currently a very active field of research. | ||
Transposons are found in all major branches of life. They may or may not have originated in the [[common descent|last universal common ancestor]], or arisen independently multiple times, or perhaps arisen once and then spread to other kingdoms by [[horizontal gene transfer]]. While transposons may confer some benefits on their hosts, they are generally considered to be [[selfish DNA]] [[parasite]]s that live within the genome of cellular organisms. In this way, they are similar to [[virus]]es. Viruses and transposons also share features in their genome structure and biochemical abilities, leading to speculation that they share a common ancestor. | Transposons are found in all major branches of life. They may or may not have originated in the [[common descent|last universal common ancestor]], or arisen independently multiple times, or perhaps arisen once and then spread to other kingdoms by [[horizontal gene transfer]]. While transposons may confer some benefits on their hosts, they are generally considered to be [[selfish DNA]] [[parasite]]s that live within the genome of cellular organisms. In this way, they are similar to [[virus]]es. Viruses and transposons also share features in their genome structure and biochemical abilities, leading to speculation that they share a common ancestor. | ||
Line 79: | Line 85: | ||
Transposons are also a widely used tool for mutagenesis in ''[[Drosophila melanogaster]]'', and a wide variety of [[bacterium|bacteria]] to study gene function. See: [[transposons as a genetic tool]]. | Transposons are also a widely used tool for mutagenesis in ''[[Drosophila melanogaster]]'', and a wide variety of [[bacterium|bacteria]] to study gene function. See: [[transposons as a genetic tool]]. | ||
== | ==References== | ||
===Notes=== | |||
<div class="references-small"> | |||
<references /> | |||
==Further reading== | ===Further reading=== | ||
* Bennetzen, J. L., 2000 Transposable element contributions to plant gene and genome evolution. Plant Molecular Biology 42: 251–269, 2000. | * Bennetzen, J. L., 2000 Transposable element contributions to plant gene and genome evolution. Plant Molecular Biology 42: 251–269, 2000. | ||
* Kidwell, M.G. (2005). Transposable elements. In ''[[The Evolution of the Genome]]'' (ed. T.R. Gregory), pp. 165-221. Elsevier, San Diego. | * Kidwell, M.G. (2005). Transposable elements. In ''[[The Evolution of the Genome]]'' (ed. T.R. Gregory), pp. 165-221. Elsevier, San Diego. | ||
Line 91: | Line 97: | ||
* Snyder, L. and Champness, W. (2003) Transpositon and site-specific recombination, Chapter 9 In Snyder, L. and Champness, W. ''Molecular Genetics of Bacteria''. 2nd. Edition. ASM Press, Washington, DC | * Snyder, L. and Champness, W. (2003) Transpositon and site-specific recombination, Chapter 9 In Snyder, L. and Champness, W. ''Molecular Genetics of Bacteria''. 2nd. Edition. ASM Press, Washington, DC | ||
== | ===See also=== | ||
*[[Mobile DNA]] | |||
*[[Insertion sequence]] | |||
*[[Barbara McClintock]] | |||
== External links == | ===External links=== | ||
* [http://www.maxanim.com/genetics/Transposition/Transposition.htm Mechanism of Transposition] (Animation) | * [http://www.maxanim.com/genetics/Transposition/Transposition.htm Mechanism of Transposition] (Animation) | ||
* [http://users.rcn.com/jkimball.ma.ultranet/BiologyPages/T/Transposons.html Kimball's Biology Pages: Transposons] | * [http://users.rcn.com/jkimball.ma.ultranet/BiologyPages/T/Transposons.html Kimball's Biology Pages: Transposons] | ||
* [http://www.newscientist.com/article.ns?id=mg19025565.500&feedId=online-news_rss20 A possible connection between aberrant reinsertions and lymphoma] - New Scientist | * [http://www.newscientist.com/article.ns?id=mg19025565.500&feedId=online-news_rss20 A possible connection between aberrant reinsertions and lymphoma] - New Scientist | ||
* [http://newton.nap.edu/html/biomems/bmcclintock.html BIOGRAPHICAL MEMOIRS National Academy of Sciences: Barbara McClintock, June 16, 1902 — September 2, 1992 By Nina V. Fedoroff] | |||
</div> | |||
<br/> | |||
Latest revision as of 07:29, 9 June 2009

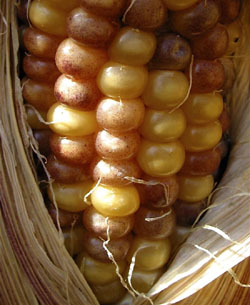
Transposons, also called "jumping genes", "mobile DNA" or "mobile genetic elements", are blocks of conserved DNA that can occasionally move to different positions within the chromosomes of a cell. The insertion of a transposon in a new site is called transposition. It can result in DNA duplication and in further multiplication of the original DNA sequence. The transposition process can cause mutations at the site of DNA insertion, and can cause increases in the total amount of DNA in a genome. Transposons were first discovered by Barbara McClintock in 1942-1948 [2], and she was eventually awarded the Nobel Prize in 1983 for this discovery [3].
Transposons insert at new locations in DNA by enzymatic breakage and reunion of DNA, a process called DNA recombination. The distinctive feature of all transposon movement is that there is no requirement for extensive DNA sequence similarity between the initial DNA region containing the transposon and the final location (that is to say, extensive base pair complementarity), and the process of transposon insertion at a new location is considered to be non-homologous recombination to distinguish it from recombination of similar DNA sequences participating in homologous recombination.
There are a variety of mobile genetic elements, and they can be broadly classified by their mechanism of transposition. Class I mobile genetic elements, or retrotransposons, move in the genome by being transcribed to RNA and then transcribed back to DNA catalyzed by the enzyme reverse transcriptase, while class II mobile genetic elements move directly from one position to another within the genome using a transposase enzyme catalyst to mobilize the DNA without involving an RNA intermediate.
For researchers, transposons are a very useful method to alter DNA inside of a living organism.
Transposons make up a large fraction of the total genome of many animals and plants which is evident through the C-values of eukaryotic species. As an example, about 45% of the human genome is composed of transposons and their defunct remnants [4].
Types of transposons
Transposons are classified into three main classes based on their mechanism of transposition.
Class I: Retrotransposons
Retrotransposons work by copying themselves and pasting copies back into the genome in multiple places. Initially, retrotransposons copy themselves to RNA (transcription) but, in addition to being translated, the RNA is copied into DNA by a reverse transcriptase (often coded by the transposon itself) and inserted back into the genome.
Retrotransposons behave very similarly to retroviruses, such as HIV, giving a clue to the evolutionary origins of such viruses. Retrotransposons are common in eukaryotic organism s(for instance maize, humans), but are rarely found in bacteria. They are present in fungi.
There are four main classes of retrotransposons:
- Viral superfamily: similar to retroviruses, have long terminal repeats (LTRs), encode reverse transcriptase (to reverse transcribe RNA into DNA).
- LINES: encode reverse transcriptase (to reverse transcribe RNA into DNA), lack LTRs, transcribed by RNA polymerase II.
- SINES: short elements that do encode reverse transcriptase but are otherwise similar to LINEs. Include the human Alu elements.
- Nonviral superfamily: do not code for reverse transcriptase, transcribed by RNA polymerase III.
Class II:DNA transposons
The major difference of Class II transposons from retrotransposons is that their transposition mechanism does not involve an RNA intermediate. Class II transposons usually move by cut and paste, rather than copy and paste, using the transposase enzyme. Different types of transposase work in different ways. Some can bind to any part of the DNA molecule, and the target site can therefore be anywhere, while others bind to specific sequences. Transposase makes a staggered cut at the target site producing sticky ends, cuts out the transposon and ligates it into the target site. A DNA polymerase fills in the resulting gaps from the sticky ends and DNA ligase closes the sugar-phosphate backbone. This results in target site duplication and the insertion sites of DNA transposons may be identified by short direct repeats (a staggered cut in the target DNA filled by DNA polymerase) which flank the inverted repeats that are part of the transposon itself (and which are important for the transposon excision by transposase).
Not all DNA transposons transpose through cut and paste mechanism. In some cases replicative transposition is observed in which transposon replicates itself to a new target site without being excised from its original site. In this process a cointegrate structure is formed containing the donor and target elements covalently connected to one-another, and in this replicative transposition resembles homologous recombination. Examples of transposons that use replicative transposition include bacteriophage Mu, Tn3 and IS1.
Both class I and class II of transposons may lose their ability to synthesize reverse transcriptase or transposase through mutation, yet continue to jump through the genome because other transposons are still producing the necessary enzyme. Transposons that themselves encode an ability to move to new locations are called autonomous transposons.
Class III: Miniature inverted-repeat transposable elements
MITEs are sequences of about 400 base pairs and 15 base pair inverted repeats that vary very little. They are found in their thousands in the genomes of both plants and animals (over 100,000 were found in the rice genome). MITEs are too small to encode any proteins.
Examples
- The first transposons were discovered in maize (Zea mays), (aka corn) by Barbara McClintock in the 1940s, for which she was awarded the Nobel Prize in 1983. She noticed the results of insertions, deletions, and translocations, caused by these transposons. These changes in the genome could, for example, lead to a change in the color of corn kernels. About 50% of the total genome of maize consists of transposons. The Ac/Ds system McClintock described are class II transposons [5].
- One family of transposons in the fruit fly Drosophila melanogaster are called P elements. They seem to have first appeared in the species only in the middle of the twentieth century. Within 50 years, they have spread through every population of the species. Artificial P elements can be used to insert genes into Drosophila by injecting the embryo. For the use of P elements as a genetic tool see: "transposons as a genetic tool".
- The simplest form of bacterial transposon are insertion sequences (IS) which are about 1000 base pairs long and consist largely of inverted DNA repeats flanking a transposase gene. Other more complex transposons in bacteria usually carry an additional gene for function other than transposition---often for antibiotic resistance. They can consist of two IS elements flanking a gene for antibiotic resistance [6]. IS elements were first discovered in the gal gene of Escherichia coli by James Shapiro in 1968 [7].
- Stimulated by Shapiro's 1968 report, follow-up molecular biology research on transposon involvement in numerous mobile DNA related phenomena in bacteria, plants and insects[8] finally led to Barbara McClintock's earlier discoveries with maize being given wide acclaim among biologists.
- In bacteria, transposons can jump from chromosomal DNA to plasmid DNA and back, allowing for the transfer and permanent addition of genes such as those encoding antibiotic resistance (multi-antibiotic resistant bacterial strains can be generated in this way). Bacterial transposons of this type belong to the Tn family.
- Mu phage in the bacterium Escherichia coli is a well known example of replicative transposition. It can exist as a prophage inserted like a transposon in the E. coli genome. Its replication by transposition mechanism leaves a copy of the prophage at the original location during transposition.[9]
- The most common form of transposon in humans is the Alu sequence, a form of SINE. The Alu sequence is approximately 300 bases long and about 1000 copies of Alu can be found in the human genome [10].
Transposons causing diseases
Transposons are mutagens. They can damage the genome of their host cell in different ways:
- A transposon or a retroposon that inserts itself into a functional gene will most likely disable that gene.
- After a transposon leaves a gene, the resulting gap will probably not be repaired correctly.
- Multiple copies of the same sequence, such as Alu sequences can hinder precise chromosomal pairing during mitosis, resulting in unequal crossovers, one of the main reasons for chromosome duplication [11] [12].
Diseases that are often caused by transposons include hemophilia A [13] and B [14], severe combined immunodeficiency[15], porphyria[16], predisposition to cancer[17] [18], and possibly Duchenne muscular dystrophy[19].
Additionally, many transposons contain promoters which drive transcription of their own transposase. These promoters can cause aberrant expression of linked genes, causing disease or mutant phenotypes.
Evolution of transposons
The evolution of transposons and their effect on genome evolution is currently a very active field of research.
Transposons are found in all major branches of life. They may or may not have originated in the last universal common ancestor, or arisen independently multiple times, or perhaps arisen once and then spread to other kingdoms by horizontal gene transfer. While transposons may confer some benefits on their hosts, they are generally considered to be selfish DNA parasites that live within the genome of cellular organisms. In this way, they are similar to viruses. Viruses and transposons also share features in their genome structure and biochemical abilities, leading to speculation that they share a common ancestor.
Since excessive transposon activity can destroy a genome, many organisms seem to have developed mechanisms to reduce transposition to a manageable level. Bacteria may undergo high rates of gene deletion as part of a mechanism to remove transposons and viruses from their genomes while eukaryotic organisms may have developed the RNA interference (RNAi) mechanism as a way of reducing transposon activity. In the nematode Caenorhabditis elegans, some genes required for RNAi also reduce transposon activity.
Transposons may have been co-opted by the vertebrate immune system as a means of producing antibody diversity. The V(D)J recombination system operates by a mechanism similar to that of transposons.
Evidence exists that transposable elements may act as mutators in bacteria and other asexual organisms.
Transposons in science
Transposons were first discovered in the plant maize. Likewise, the first transposon to be molecularly isolated was from a plant (Snapdragon). Appropriately, transposons have been an especially useful tool in plant molecular biology. Researchers use transposons as a means of mutagenesis. In this context, a transposon jumps into a gene and produces a mutation. The presence of the transposon provides a straightforward means of identifying the mutant allele, relative to chemical mutagenesis methods.
Sometimes the insertion of a transposon into a gene can disrupt that gene's function in a reversible manner; transposase mediated excision of the transposon restores gene function. This produces plants in which neighboring cells have different genotypes. This feature allows researchers to distinguish between genes that must be present inside of a cell in order to function (cell-autonomous) and genes that produce observable effects in cells other than those where the gene is expressed.
Transposons are also a widely used tool for mutagenesis in Drosophila melanogaster, and a wide variety of bacteria to study gene function. See: transposons as a genetic tool.
References
Notes
- ↑ Transposon Silencing Keeps Jumping Genes in Their Place Gross L PLoS Biology Vol. 4, No. 10, e353 doi:10.1371/journal.pbio.0040353
- ↑ The Barbara McClintock Papers, National Library of Medicine: McClintock, B. "Cytogenetic Studies of Maize and Neurospora." Carnegie Institution of Washington Yearbook 44, (1945): 108-112. McClintock, B. "Maize Genetics." Carnegie Institution of Washington Yearbook 45, (1946): 176-186. McClintock, B. "Mutable Loci in Maize." Carnegie Institution of Washington Yearbook 47, (1948): 155-169.
- ↑ BIOGRAPHICAL MEMOIRS National Academy of Sciences: Barbara McClintock June 16, 1902 — September 2, 1992 By Nina V. Fedoroff
- ↑ International Human Genome Sequencing Consortium Initial sequencing and analysis of the human genome NATURE VOL 409 15 FEBRUARY 2001, p 860-921
- ↑ Bennetzen, J. L., 2000 Transposable element contributions to plant gene and genome evolution. Plant Molecular Biology 42: 251–269, 2000.
- ↑ Snyder, L. and Champness, W. (2003)Transposon and site-specific recombination, Chapter 9 In Snyder, L. and Champness, W. Molecular Genetics of Bacteria. 2nd. Edition. ASM Press, Washington, DC.
- ↑ Shapiro, J.A. (1968). Mutations caused by insertion of genetic material into the galactose operon of Escherichia coli. Journal of Molecular Biology 40, p93-105.
- ↑ Berg, D. E. and Howe, M. M. Mobile DNA ASM Press, Washington DC.
- ↑ Ljungquist, E. and Bukhati, A. I.(1977) State of prophage Mu upon induction. PNAS USA 74, p3143-3147).
- ↑ International Human Genome Sequencing Consortium Initial sequencing and analysis of the human genome NATURE VOL 409 15 FEBRUARY 2001, p 860-921
- ↑ Abeysinghe SS, Chuzhanova N, Krawczak M, Ball EV, Cooper DN.(2003) Translocation and gross deletion breakpoints in human inherited disease and cancer I: Nucleotide composition and recombination-associated motifs. Hum Mutat. 2003 Sep;22(3):229-44.
- ↑ Kolomietz E, Meyn MS, Pandita A, Squire JA. (2002) The role of Alu repeat clusters as mediators of recurrent chromosomal aberrations in tumors.Genes Chromosomes Cancer. 2002 Oct;35(2):97-112.
- ↑ Ganguly A, Dunbar T, Chen P, Godmilow L, Ganguly T. (2003) Exon skipping caused by an intronic insertion of a young Alu Yb9 element leads to severe hemophilia A. Hum Genet. 2003 Sep;113(4):348-52. Epub 2003 Jul 12. PMID: 12884004
- ↑ Li X, Scaringe WA, Hill KA, Roberts S, Mengos A, Careri D, Pinto MT, Kasper CK, Sommer SS.(2001) Frequency of recent retrotransposition events in the human factor IX gene.Hum Mutat. 2001 Jun;17(6):511-9.
- ↑ Markert ML, Hutton JJ, Wiginton DA, States JC, Kaufman RE. (1988) Adenosine deaminase (ADA) deficiency due to deletion of the ADA gene promoter and first exon by homologous recombination between two Alu elements. J Clin Invest. 1988 May;81(5):1323-7.
- ↑ Mustajoki S, Ahola H, Mustajoki P, Kauppinen R.(1999) Insertion of Alu element responsible for acute intermittent porphyria.Hum Mutat. 1999;13(6):431-8.PMID: 10408772
- ↑ Teugels E, De Brakeleer S, Goelen G, Lissens W, Sermijn E, De Greve J.(2005) De novo Alu element insertions targeted to a sequence common to the BRCA1 and BRCA2 genes.Hum Mutat. 2005 Sep;26(3):284.
- ↑ Gibbons R, Dugaiczyk A.(2005) Phylogenetic roots of Alu-mediated rearrangements leading to cancer. Genome. 2005 Feb;48(1):160-7.
- ↑ McNaughton JC, Hughes G, Jones WA, Stockwell PA, Klamut HJ, Petersen GB.(1997) The evolution of an intron: analysis of a long, deletion-prone intron in the human dystrophin gene.Genomics. 1997 Mar 1;40(2):294-304.
Further reading
- Bennetzen, J. L., 2000 Transposable element contributions to plant gene and genome evolution. Plant Molecular Biology 42: 251–269, 2000.
- Kidwell, M.G. (2005). Transposable elements. In The Evolution of the Genome (ed. T.R. Gregory), pp. 165-221. Elsevier, San Diego.
- Craig NL, Craigie R, Gellert M, and Lambowitz AM (ed.) (2002) Mobile DNA II, ASM Press, Washington, DC.
- Lewin B (2000) Genes VII, Oxford University Press.
- Snyder, L. and Champness, W. (2003) Transpositon and site-specific recombination, Chapter 9 In Snyder, L. and Champness, W. Molecular Genetics of Bacteria. 2nd. Edition. ASM Press, Washington, DC
See also
External links