Michael Faraday: Difference between revisions
imported>Daniel Mietchen m (broken intrawiki link) |
imported>J. Noel Chiappa (Clarify plagiarism stuff, move some chemistry into bio, add material on his influenceon field theory) |
||
Line 1: | Line 1: | ||
{{subpages}} | {{subpages}} | ||
'''Michael Faraday''' (September 22, 1791 – August 25, 1867) was an English physicist and chemist | '''Michael Faraday''' (September 22, 1791 – August 25, 1867) was an English physicist and chemist who is one of the most influential scientists of all time.<ref>Simmons, John G. ''The Scientific 100: A Ranking of the Most Influential Scientists, Past and Present''</ref> His most important contributions, and best known work, were on the affiliated phenomena of [[electricity]] and [[magnetism]], but he also made very significant contributions in chemistry. | ||
Faraday was | Faraday was principally an experimentalist; in fact, he has been described as the "best experimentalist in the history of science".<ref>[http://www.bath.ac.uk/news/2006/10/25/gulp-ford251006.html] Press Release, University of Bath, 25 October 2006</ref> He himself did not know any advanced mathematics, and as a result he has been likened to [[Moses]], in that he brought the scientific fields he worked in to a place he himself could not enter. | ||
In | However, both his contributions to science, and his impact on the world, are nonetheless vast: his scientific discoveries underlie significant areas of modern physics and chemistry, and the technology which evolved from his work is even more widespread. His discoveries in [[electromagnetism]] laid the groundwork for the engineering work in the late 1800s by people such as [[Thomas Edison|Edison]], [[Werner von Siemens|Siemens]], [[Nikola Tesla|Tesla]] and [[George Westinghouse|Westinghouse]], <!-- Those 4 are listed in alphabetic order to avoid a debate over the relative magnitude of their contributions. --> which brought about the [[electrification]] of industrial societies, and his work in [[electrochemistry]] is now widely used in the field of [[chemical engineering]]. | ||
In [[physics]], he was one of the first to explore the ways in which electricity and magnetism are associated. In 1821, shortly after [[Hans Christian Oersted|Oersted]] first discovered that electricity and magnetism were conjoined, Faraday published his work on what he called ''electromagnetic rotation'' (the principle behind the [[electric motor]]). In 1831, Faraday discovered [[electromagnetic induction]], the principle behind the [[electric generator]] and [[electric transformer]]. His ideas about electrical and magnetic [[fields]], and the nature of fields in general, laid the groundwork for later work in this area (such as [[Maxwell's equations]]), and fields of the type he envisaged are a key concept in today's physics. | |||
In chemistry, he succeeded in liquifying gasses, making clear that a gas could exist in more than one phase; he also discovered [[benzene]]. Perhaps his biggest contribution was in virtually founding electrochemistry, including popularizing terminology such as [[anode]], [[cathode]], [[electrode]], and [[ion]]. <ref>Simmons, John G. ''The Scientific 100: A Ranking of the Most Influential Scientists, Past and Present'', pp. 60, 62 for gasses and electrochemistry, respectively. </ref> | |||
[[Image:Faraday.jpg|thumb|250px|Michael Faraday. Photograph by John Watkins, ca. 1861]] | [[Image:Faraday.jpg|thumb|250px|Michael Faraday. Photograph by John Watkins, ca. 1861]] | ||
Line 44: | Line 48: | ||
In the experiment he created to investigate these concepts, he managed to demonstrate both of these effects (''see the section [[#Electromagnetic rotation|below]] on electromagnetic rotation for more details''). In one part of the experiment a steady [[direct current]] moved a magnet in circles; in another a similar current caused a wire to steadily rotate. These discoveries, made in the second half of 1821, are the basis of all electric motors, and it brought Faraday instant world fame. | In the experiment he created to investigate these concepts, he managed to demonstrate both of these effects (''see the section [[#Electromagnetic rotation|below]] on electromagnetic rotation for more details''). In one part of the experiment a steady [[direct current]] moved a magnet in circles; in another a similar current caused a wire to steadily rotate. These discoveries, made in the second half of 1821, are the basis of all electric motors, and it brought Faraday instant world fame. | ||
Unfortunately, the achievement | Unfortunately, the achievement started a rift between Davy and Faraday, which a later incident over work on liquifying chlorine was to exacerbate. Davy was apparently of the opinion that Faraday had relied on some work done by Davy and [[William Wollaston]], without properly acknowledging their contribution. In April 1821, Wollaston, after hearing of Oersted's discovery, had visited the Royal Institution, and in collaboration with Davy had tried — in vain — something similar to what Faraday managed to do later that year.<ref> Frank A. J. L. James, "Faraday, Michael (1791–1867)", ''Oxford Dictionary of National Biography,'' Sept 2004; online edn, Jan 2008 </ref> | ||
==Further scientific accomplishments== | |||
Also in 1821, Faraday married Sarah Barnard, another Sandemanian, on June 12. Although they never had children, Sarah was a steadying influence in Faraday's life; she was a warm and charming person filled with maternal feelings which, in the absence of children, she lavished upon her husband and her nieces. The couple lived "above the store", at the top of the Royal Institute building, until 1862. | |||
Following a suggestion of Davy, Faraday managed to unexpectedly liquify [[chlorine]] in March 1823 — to the further displeasure of Davy, who felt that he deserved partial credit for this discovery, since he had suggested the problem to Faraday.<ref> Frank A. J. L. James, "Faraday, Michael (1791–1867)", ''Oxford Dictionary of National Biography,'' Sept 2004; online edn, Jan 2008 </ref> | |||
Faraday became a [[Fellow of the Royal Society]] in 1824, with one vote against him; it is believed that this was Davy's. (If this is so, Wollaston must have voted in favor of Faraday.) The usual explanation was that this resulted from their prior disputes, but it may have been simply because of Davy's public stance against nepotism.<ref>Farndon, John et al. The Great Scientists: From Euclid to Stephen Hawking, Metro Books, New York (2007) pp. 82 </ref> | |||
Apparently as a result of his disputes with Davy, he spent much of the 1820s working on less important problems, but he still managed a few discoveries. In 1825, he discovered [[benzene]], which he called ''bicarburet of hydrogen''. He isolated it from a liquid obtained in the production of oil gas. At the same time he did research on glass that, however, did not have much result; but he found the recipe for ''heavy glass''. | |||
In 1825, Faraday became director of the Laboratory of the Royal Institution and, beginning in 1826, he revived the tradition of popular lectures at the Institution, giving many himself. For many years, around Christmas he and others delivered a short lecture series especially for children, which attracted an audience from the upper social classes of London. | In 1825, Faraday became director of the Laboratory of the Royal Institution and, beginning in 1826, he revived the tradition of popular lectures at the Institution, giving many himself. For many years, around Christmas he and others delivered a short lecture series especially for children, which attracted an audience from the upper social classes of London. | ||
Line 57: | Line 70: | ||
In 1833 Faraday was honored by a [[Fullerian Professor]]ship of chemistry at the Royal Institution; the chair was especially created for him, and still exists today. (One of Faraday's biographers, J. H. Gladstone, later held the Fullerian professorship for three years.) | In 1833 Faraday was honored by a [[Fullerian Professor]]ship of chemistry at the Royal Institution; the chair was especially created for him, and still exists today. (One of Faraday's biographers, J. H. Gladstone, later held the Fullerian professorship for three years.) | ||
In the 1830s Faraday also did very important work in electrochemistry, and formulated two laws in that area which now carry his name. | |||
During the following years he developed the idea of electric and magnetic ''force lines'', (later called ''fields'' by [[William Thomson]], Lord Kelvin). Some details of his concepts contradicted the then widely-held belief that electromagnetic effects involved ''instantaneous action at a distance''. Because of his background, Faraday hardly knew any mathematics, and his intuitive ideas were qualitative and non-mathematical. Most of the contemporary physicists, who generally were well versed in the mathematical formulation of Sir [[Isaac Newton]]'s mechanics, in which action at a distance plays an important role, frowned upon Faraday's ideas. <!-- I think I have put this correctly. I had a quick look for something that talks about Faraday's views on 'instantanous', but didn't quickly find anything. --> | During the following years he developed the idea of electric and magnetic ''force lines'', (later called ''fields'' by [[William Thomson]], Lord Kelvin). Some details of his concepts contradicted the then widely-held belief that electromagnetic effects involved ''instantaneous action at a distance''. Because of his background, Faraday hardly knew any mathematics, and his intuitive ideas were qualitative and non-mathematical. Most of the contemporary physicists, who generally were well versed in the mathematical formulation of Sir [[Isaac Newton]]'s mechanics, in which action at a distance plays an important role, frowned upon Faraday's ideas. <!-- I think I have put this correctly. I had a quick look for something that talks about Faraday's views on 'instantanous', but didn't quickly find anything. --> | ||
However, at the end of Faraday's life his ideas were cast into a concise and elegant mathematical form by [[James Clerk Maxwell]]. | However, at the end of Faraday's life his ideas were cast into a concise and elegant mathematical form by [[James Clerk Maxwell]]. 'Maxwell's equations', as they are now known, are today still the accepted form of the theory of electromagnetism. Among many other important results, they show that visible [[light]] is an [[electromagnetic wave]], as are [[radio waves]], [[microwaves]], [[infrared radiation|infrared]] and [[gamma radiation]], which are collectively known as [[electromagnetic radiation]]. | ||
Faraday was strong physically, but suffered occasionally from headaches, memory lapses, and bouts of depression. These symptoms increased in severity and frequency until in 1840, at the age of forty-nine, Faraday had a major [[nervous breakdown]]. For four years he was hardly able to work. | Faraday was strong physically, but suffered occasionally from headaches, memory lapses, and bouts of depression. These symptoms increased in severity and frequency until in 1840, at the age of forty-nine, Faraday had a major [[nervous breakdown]]. For four years he was hardly able to work. | ||
However, by 1845 he had recovered, and started his research activities again. Because he believed strongly in the unity of forces, he again investigated the effect of magnetic fields on light, an area he had previously investigated without success, and made another major discovery in 1845. Acting on a suggestion by William Thomson (later [[Lord Kelvin]]), he discovered such a connection; the [[polarization]] of [[polarized light]] is affected by a magnetic field. This ability of magnetism to affect light is now known as the [[Faraday effect]]. In the same series of experiments, he also discovered [[diamagnetism]]. | However, by 1845 he had recovered, and started his research activities again. Because he believed strongly in the unity of forces, he again investigated the effect of magnetic fields on light, an area he had previously investigated without success, and made another major discovery in 1845. Acting on a suggestion by William Thomson (later [[Lord Kelvin]]), he discovered such a connection; the [[polarization plane]] of [[polarized light]] is affected by a magnetic field. This ability of magnetism to affect light is now known as the [[Faraday effect]]. In the same series of experiments, he also discovered [[diamagnetism]]. | ||
===Later life=== | ===Later life=== | ||
Line 98: | Line 113: | ||
Since the iron ring passes through coil ''B'', then as long as the current is flowing through coil ''A'', ''B'' is wound around a magnet as well. Faraday had discovered that changes in the strength of the magnetic field of the electromagnet (which occur when switching it on and off) produces a current in the wire connected to coil ''B'' (which, remember, is not in electric contact with ''A''). The proof that a current was running through ''B'' was the movement of the magnetic needle; it was known from Oersted's work that a current flowing in a wire will move a nearby magnetic needle. | Since the iron ring passes through coil ''B'', then as long as the current is flowing through coil ''A'', ''B'' is wound around a magnet as well. Faraday had discovered that changes in the strength of the magnetic field of the electromagnet (which occur when switching it on and off) produces a current in the wire connected to coil ''B'' (which, remember, is not in electric contact with ''A''). The proof that a current was running through ''B'' was the movement of the magnetic needle; it was known from Oersted's work that a current flowing in a wire will move a nearby magnetic needle. | ||
Within a few weeks he further investigated this effect by setting up a cylindrical coil | Within a few weeks he further investigated this effect by setting up a cylindrical coil made with 70 meters of wire, and arranging to rapidly move a permanent cylindrical magnet of about 22 centimeters length up and down inside the coil. He then was able to observe an electric current in the coil, ''induced'' by the moving magnet. The direction of the current produced in the coil when the magnet was moved into the coil was the reverse of that produced when it was then pulled out. This important experiment proved that moving a wire through a magnetic field produced a current in the wire (because it was logically equivalent to holding the magnet still, and moving the coil instead). | ||
He later succeeding in generating a current by rotating a copper disk between the poles of a large horseshoe magnet; the disk had one wire fixed to its center, and another made sliding contact along the edge of the disk. This was the first generator, and all generators today are its descendants. | |||
Faraday called this effect electromagnetic induction, and he was fascinated by the symmetry revealed by the effect that he now had discovered. Previously, it had been known that a moving electric charge (i.e. a current) produced a magnetic field; now it had been shown that a moving magnet produced an electric field (it is this field which causes the current to flow). | Faraday called this effect electromagnetic induction, and he was fascinated by the symmetry revealed by the effect that he now had discovered. Previously, it had been known that a moving electric charge (i.e. a current) produced a magnetic field; now it had been shown that a moving magnet produced an electric field (it is this field which causes the current to flow). | ||
Magnetic induction is of extreme importance in modern industrial society, because it is the principle behind [[electric generators]] and [[transformers]]. | Magnetic induction is of extreme importance in modern industrial society, because it is the principle behind [[electric generators]] and [[transformers]]. | ||
<!-- | <!-- (The importance of the latter is that without simple, passive device to alter the [[voltage]] of electricity, economical and safe distribution of electric power would not have been possible.) | ||
(The importance of the latter is that without simple, passive device to alter the [[voltage]] of electricity, economical and safe distribution of electric power would not have been possible.) | I would save this statement for an article on transformers (PW). | ||
Well, I put it in because I thought it would help our average readers understand the importance of this discovery. But I don't feel strongly that it has to be here. [jnc] | |||
--> | --> | ||
===Chemistry=== | ===Chemistry=== | ||
The basic setup of electrochemistry is simple: it consists of a vessel containing an [[electrolyte]], which is a solution of charged particles ([[ion]]s). Since this solution is neutral in total, the total charge carried by the positive particles (cations) is he same (in absolute value) as the total charge carried by the negative particles (anions). Two [[electrodes]] are dipped into the electrolyte. Outside the vessel a direct current (DC) runs from the negatively charged electrode (the cathode) to the positively charged electrode (the anode). Inside the vessel cations move to the cathode, pick up electrons—which carry negative charge—so that the cations are neutralized and are deposited on the cathode (or if the neutral species is gaseous, it escapes from the cathode in the form of gas). At the anode the opposite happens: anions lose their excess electrons and are deposited. Outside the vessel the electrons run from the anode to the cathode (by definition they run in direction opposite to the DC). Faraday's first law states that the amount of substance deposited on the electrodes is proportional to the amount of current that passed through the electrodes. In the case of a steady current this amount is equal to the time that the current has been running multiplied by the strength of the current. A constant proportional to this amount is called after Faraday—[[Faraday's constant]]. Faraday's second law of electrochemistry is a recognition of the fact that anions and cations may carry one, two, three, etc. [[elementary charge]]s, so that it takes one, two, three, etc., electrons, respectively, to neutralize the cation, while the anion must lose one, two, three, etc. electron to become neutral. These differently charged ions will all give the deposition of one atom only, so that, e.g., twice as much electricity is needed to generate an atom from a doubly charged ion as from a singly charged ion. Faraday was the first to see these facts clearly, moreover, he introduced the corresponding terminology (electrolyte, electrode, anode, cathode, ion, anion, and cation) derived from old Greek, helped by his friend [[William Whewell]] of Trinity College, Cambridge, who knew Greek. | The basic setup of electrochemistry is simple: it consists of a vessel containing an [[electrolyte]], which is a solution of charged particles ([[ion]]s). Since this solution is neutral in total, the total charge carried by the positive particles (cations) is he same (in absolute value) as the total charge carried by the negative particles (anions). Two [[electrodes]] are dipped into the electrolyte. Outside the vessel a direct current (DC) runs from the negatively charged electrode (the cathode) to the positively charged electrode (the anode). Inside the vessel cations move to the cathode, pick up electrons—which carry negative charge—so that the cations are neutralized and are deposited on the cathode (or if the neutral species is gaseous, it escapes from the cathode in the form of gas). At the anode the opposite happens: anions lose their excess electrons and are deposited. Outside the vessel the electrons run from the anode to the cathode (by definition they run in direction opposite to the DC). Faraday's first law states that the amount of substance deposited on the electrodes is proportional to the amount of current that passed through the electrodes. In the case of a steady current this amount is equal to the time that the current has been running multiplied by the strength of the current. A constant proportional to this amount is called after Faraday—[[Faraday's constant]]. Faraday's second law of electrochemistry is a recognition of the fact that anions and cations may carry one, two, three, etc. [[elementary charge]]s, so that it takes one, two, three, etc., electrons, respectively, to neutralize the cation, while the anion must lose one, two, three, etc. electron to become neutral. These differently charged ions will all give the deposition of one atom only, so that, e.g., twice as much electricity is needed to generate an atom from a doubly charged ion as from a singly charged ion. Faraday was the first to see these facts clearly, moreover, he introduced the corresponding terminology (electrolyte, electrode, anode, cathode, ion, anion, and cation) derived from old Greek, helped by his friend [[William Whewell]] of Trinity College, Cambridge, who knew Greek. | ||
Line 133: | Line 149: | ||
===External links=== | ===External links=== | ||
* [http://www-groups.dcs.st-and.ac.uk/~history/Biographies/Faraday.html Faraday biography in the MacTutor History of Mathematics Archive] | * [http://www-groups.dcs.st-and.ac.uk/~history/Biographies/Faraday.html Faraday biography] in the MacTutor History of Mathematics Archive at the School of Mathematical and Computational Sciences at the University of St Andrews | ||
* [http://chem.ch.huji.ac.il/history/faraday.htm Michael Faraday] biography at the Institute of Chemistry at the Hebrew University of Jerusalem | |||
* [http://www.rigb.org/heritage/faradaypage.jsp Biography of Faraday] (a fairly extensive biography by Dr. Frank A. J. L. James) | * [http://www.rigb.org/heritage/faradaypage.jsp Biography of Faraday] (a fairly extensive biography by Dr. Frank A. J. L. James) | ||
* [http://www.rigb.org/heritage/downloads/Guides%20to%20the%20RI%20no.1.pdf History of the Royal Institution of Great Britain] (by Dr. Frank A.J.L. James; has much information on Faraday) | * [http://www.rigb.org/heritage/downloads/Guides%20to%20the%20RI%20no.1.pdf History of the Royal Institution of Great Britain] (by Dr. Frank A.J.L. James; has much information on Faraday) |
Revision as of 13:35, 25 April 2008

Michael Faraday (September 22, 1791 – August 25, 1867) was an English physicist and chemist who is one of the most influential scientists of all time.[1] His most important contributions, and best known work, were on the affiliated phenomena of electricity and magnetism, but he also made very significant contributions in chemistry.
Faraday was principally an experimentalist; in fact, he has been described as the "best experimentalist in the history of science".[2] He himself did not know any advanced mathematics, and as a result he has been likened to Moses, in that he brought the scientific fields he worked in to a place he himself could not enter.
However, both his contributions to science, and his impact on the world, are nonetheless vast: his scientific discoveries underlie significant areas of modern physics and chemistry, and the technology which evolved from his work is even more widespread. His discoveries in electromagnetism laid the groundwork for the engineering work in the late 1800s by people such as Edison, Siemens, Tesla and Westinghouse, which brought about the electrification of industrial societies, and his work in electrochemistry is now widely used in the field of chemical engineering.
In physics, he was one of the first to explore the ways in which electricity and magnetism are associated. In 1821, shortly after Oersted first discovered that electricity and magnetism were conjoined, Faraday published his work on what he called electromagnetic rotation (the principle behind the electric motor). In 1831, Faraday discovered electromagnetic induction, the principle behind the electric generator and electric transformer. His ideas about electrical and magnetic fields, and the nature of fields in general, laid the groundwork for later work in this area (such as Maxwell's equations), and fields of the type he envisaged are a key concept in today's physics.
In chemistry, he succeeded in liquifying gasses, making clear that a gas could exist in more than one phase; he also discovered benzene. Perhaps his biggest contribution was in virtually founding electrochemistry, including popularizing terminology such as anode, cathode, electrode, and ion. [3]
Biography
The Faraday family came from the North of England; before Michael Faraday was born, his father James, a blacksmith, took his wife Margaret and two small children to the South, in search of work. The family settled briefly in Newington Butts, a borough in South London (it was then a separate village, but is now part of Southwark), where Faraday was born. The family soon moved into London itself.
His father was in poor health (he died in 1810), and Faraday grew up in poverty. The family was close, and obtained strength from their orthodox faith, the Sandemanians, a small dissident spin-off from the Presbyterian church. Faraday would stay faithful to that group for the rest of his life.
At fourteen he became an apprentice at the shop of the bookseller and bookbinder George Ribeau, and moved in with Ribeau's family; in such a household there were always books around for him to read. For instance, the third edition of the Encyclopedia Brittanica was one of the shop's bookbinding assignments, and his fascination for electricity was first stirred by reading the encyclopedia article about it.
Initial professional career
At twenty-one, nearing the end of his apprenticeship, he was given tickets for a series of four lectures on chemistry delivered by Sir Humphrey Davy at the Royal Institution, a gift from William Dance, a customer of the bookshop who was a member of the Royal Institution. These lectures, in the spring of 1812, were recorded by Faraday in careful lecture notes, which he neatly bound into a book.
By the fall of 1812 Faraday was a fully-qualified bookbinder, and moved to another firm. He did not particularly like his new job, but within a few weeks his life took a sudden turn. Davy needed help for a few days at the Royal Institution, from someone with a rudimentary knowledge of chemistry, and Faraday got the job (probably as the result of a recommendation from a customer of his first employer).
Faraday showed his lecture notes to Davy, who no doubt would have been pleased by the attention to his lectures. After the temporary job ended, Faraday had to leave the Royal Institution. However, as luck would have it, soon afterwards Davy's laboratory assistant was fired, because of a drinking problem, and the position became vacant. Not surprisingly, Faraday got the job, and started work at the Royal Institution on 1 March 1813, where he would stay for fifty-two years, until 1865.
Half a year later, Faraday was invited by Davy to accompany Lady Davy and him on a tour of continental Europe. Davy was a renowned "natural philosopher" (the name "scientist" for this profession was only coined later, by William Whewell), and had unquestioned entry to scientific circles there. Faraday accepted the invitation, but during the tour sometimes regretted that decision, because Lady Davy (who is known to have been very snobbish) treated him as a lowly servant.
Professionally, however, the tour was a great success for Faraday, because he had the chance to converse with many of the leading scientists in France, Switzerland and Italy. In addition, he saw the Alps and the Mediterranean, learned French and Italian, and became Davy's collaborator, not just his assistant.
The chemist John Hall Gladstone (1827–1902), who knew Faraday well, wrote:
This year and a half may be considered as the time of Faraday's education; it was the period of his life that best corresponds with the collegiate course of other men who have attained high distinction in the world of thought. But his University was Europe; his professors the master whom he served, and those illustrious men to whom the renown of Davy introduced the travelers. [4]
Faraday's improved abilities were recognized upon his return to the Royal Institution; he was promoted to superintendent of apparatus, and was given better rooms (he was living at the top of the Royal Institution building in Albemarle Street).
Until early in 1820, Faraday's work was mostly in chemistry, and he earned an international reputation as a good, solid chemist—but not yet a brilliant one. His reputation as a chemist received significant boosts when he managed to liquify chlorine (1823), and discovered benzene (1825).
First major discovery
In physics, his first major achievement was to show that electricity could be used to force a magnet into continual rotational motion, as long as the electricity continued to flow.[5] He also decided that if current in a wire could move a magnet, then if the magnet were held fixed, and the wire allowed to be mobile, then a current flowing in the wire should cause it to move.
In the experiment he created to investigate these concepts, he managed to demonstrate both of these effects (see the section below on electromagnetic rotation for more details). In one part of the experiment a steady direct current moved a magnet in circles; in another a similar current caused a wire to steadily rotate. These discoveries, made in the second half of 1821, are the basis of all electric motors, and it brought Faraday instant world fame.
Unfortunately, the achievement started a rift between Davy and Faraday, which a later incident over work on liquifying chlorine was to exacerbate. Davy was apparently of the opinion that Faraday had relied on some work done by Davy and William Wollaston, without properly acknowledging their contribution. In April 1821, Wollaston, after hearing of Oersted's discovery, had visited the Royal Institution, and in collaboration with Davy had tried — in vain — something similar to what Faraday managed to do later that year.[6]
Further scientific accomplishments
Also in 1821, Faraday married Sarah Barnard, another Sandemanian, on June 12. Although they never had children, Sarah was a steadying influence in Faraday's life; she was a warm and charming person filled with maternal feelings which, in the absence of children, she lavished upon her husband and her nieces. The couple lived "above the store", at the top of the Royal Institute building, until 1862.
Following a suggestion of Davy, Faraday managed to unexpectedly liquify chlorine in March 1823 — to the further displeasure of Davy, who felt that he deserved partial credit for this discovery, since he had suggested the problem to Faraday.[7]
Faraday became a Fellow of the Royal Society in 1824, with one vote against him; it is believed that this was Davy's. (If this is so, Wollaston must have voted in favor of Faraday.) The usual explanation was that this resulted from their prior disputes, but it may have been simply because of Davy's public stance against nepotism.[8]
Apparently as a result of his disputes with Davy, he spent much of the 1820s working on less important problems, but he still managed a few discoveries. In 1825, he discovered benzene, which he called bicarburet of hydrogen. He isolated it from a liquid obtained in the production of oil gas. At the same time he did research on glass that, however, did not have much result; but he found the recipe for heavy glass.
In 1825, Faraday became director of the Laboratory of the Royal Institution and, beginning in 1826, he revived the tradition of popular lectures at the Institution, giving many himself. For many years, around Christmas he and others delivered a short lecture series especially for children, which attracted an audience from the upper social classes of London.
The most famous of the Christmas lecture series, in 1848, called The Chemical History of a Candle, was published, and has since gone through innumerable editions in many languages.[9] Another well-known series directed to young persons is about various forces in nature, from 1859.[10]
Most significant technical achievement
In 1831 Faraday made what many consider his most important discovery, which is that a changing magnetic field (either from a moving magnet, or a wire moving through a non-uniform magnetic field) can 'induce' an electric current in a wire (see the section below on electromagnetic induction for more details). He named this phenomenon electromagnetic induction, and it is used today in almost all production of electricity.
In 1833 Faraday was honored by a Fullerian Professorship of chemistry at the Royal Institution; the chair was especially created for him, and still exists today. (One of Faraday's biographers, J. H. Gladstone, later held the Fullerian professorship for three years.)
In the 1830s Faraday also did very important work in electrochemistry, and formulated two laws in that area which now carry his name.
During the following years he developed the idea of electric and magnetic force lines, (later called fields by William Thomson, Lord Kelvin). Some details of his concepts contradicted the then widely-held belief that electromagnetic effects involved instantaneous action at a distance. Because of his background, Faraday hardly knew any mathematics, and his intuitive ideas were qualitative and non-mathematical. Most of the contemporary physicists, who generally were well versed in the mathematical formulation of Sir Isaac Newton's mechanics, in which action at a distance plays an important role, frowned upon Faraday's ideas.
However, at the end of Faraday's life his ideas were cast into a concise and elegant mathematical form by James Clerk Maxwell. 'Maxwell's equations', as they are now known, are today still the accepted form of the theory of electromagnetism. Among many other important results, they show that visible light is an electromagnetic wave, as are radio waves, microwaves, infrared and gamma radiation, which are collectively known as electromagnetic radiation.
Faraday was strong physically, but suffered occasionally from headaches, memory lapses, and bouts of depression. These symptoms increased in severity and frequency until in 1840, at the age of forty-nine, Faraday had a major nervous breakdown. For four years he was hardly able to work.
However, by 1845 he had recovered, and started his research activities again. Because he believed strongly in the unity of forces, he again investigated the effect of magnetic fields on light, an area he had previously investigated without success, and made another major discovery in 1845. Acting on a suggestion by William Thomson (later Lord Kelvin), he discovered such a connection; the polarization plane of polarized light is affected by a magnetic field. This ability of magnetism to affect light is now known as the Faraday effect. In the same series of experiments, he also discovered diamagnetism.
Later life
True to his Sandemanian principles, Faraday turned down the offer of a knighthood, and twice declined to become president of the Royal Society. In 1861, when he was seventy years old, he resigned from the Royal Institution, but he was asked to stay on in a nominal post, which he did until 1865.
In 1862, he and Sarah moved out from Albemarle Street into a house provided a few years earlier by Queen Victoria, at the suggestion of her husband Prince Albert. He died there on 25 August, 1867. In a characteristic display of his lifelong modesty, he turned down an offer to be buried in Westminster Abbey, as he preferred a simpler funeral and grave; he is buried in Highgate Cemetery.
Faraday's science
Electromagnetic rotation
When Faraday heard of Oersted's 1820 discovery that a steady electric current in a wire generates a cylindrical magnetic field (with the current-carrying wire as the axis of the cylinder), it occurred to him that a magnetic pole would be pushed around a circle by such a field. Hence, it would rotate forever, or at least as long as the current is flowing. He also reasoned that if a current in a wire can move a magnet, a magnet should be able to move a current-carrying wire. In 1821 he designed the apparatus shown in the figure on the right: it includes two distinct mechanisms, one for each of the two basic concepts he was working on. In one, electricity propels a moveable magnet, and in the other, a fixed magnet causes a mobile wire to move when electricity flows through it.[11]
In the vessel on the left, a strong bar magnet floats on end in a mercury bath, held in place only by a thread at its bottom. (Recall that mercury is a heavy liquid metallic element that is a very good conductor of electricity.) A fixed copper wire dips into the mercury at the top of the bath; at the bottom of the vessel, another wire also projects into the mercury.
In the vessel on the right, another bar magnet is fixed in an upright position in the middle of another bath of mercury. A conducting (copper) socket extends into the bottom of the bath, and a copper wire which hangs from a flexible joint above the mercury bath dips into the top of the bath; the joint allows the top wire to pivot relatively freely around the joint.
When a direct current is switched on in either vessel (running in through one wire, through the mercury bath, and out through the other wire), it produces motion of the mechanism in that bath. The wire in the one on the right rotates around the magnet so fast that — as described by Faraday — the eye can scarcely follow the motion; the magnet on the left rotates around the fixed wire.
Note that Faraday's setup is such that only one pole of each of the two poles of the magnets is utilized. If either the magnets is turned around (i.e., the North and South poles are interchanged), the rotation which is observed in that vessel changes direction. The same happens if the direction of the current is reversed. Faraday, who coined the term electromagnetic rotation for this effect, had in fact invented a primitive precursor to the electric motor.
Electromagnetic induction
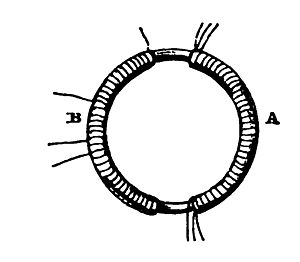
In a series of experiments performed in August 1831, Faraday, knowing that electricity can create magnetism, investigated whether the converse effect is also true; in other words, whether magnetism has an effect on an electric current.
For this, he wound two coils of insulated wire around either side of a soft iron ring of six inches external diameter; the ring itself was 7/8 inch thick. (See the illustration on the left.) The coils were not connected to each other. The coil A on the right-hand side could be connected to a battery; a copper wire attached to the coil B was led over a magnetic needle a short distance away from the ring. At the moment the battery was connected to coil A, and the current began to flow, the needle oscillated, and after a while settled into its original position. When the battery was disconnected from A, a disturbance of the needle was again observed.
When Faraday performed this experiment, it was already known that the part of the iron ring covered by coil A becomes a magnet — an electromagnet — when current runs through the coil. (The separation of the test magnetic needle was necessary to ensure that the electromagnetism of the powered coil A did not effect the magnetic needle.) The magnetic North and South poles of this electromagnet are at the beginning and the end of coil A — which end is North depends on the direction of the current in the coil A. The magnetic field produced by the electromagnet is effectively channeled from one end of it around to the other end through the iron of the ring, which is more hospitable to magnetic fields than air, a property known as magnetic permeability, thereby turning the entire ring into a magnet.
Since the iron ring passes through coil B, then as long as the current is flowing through coil A, B is wound around a magnet as well. Faraday had discovered that changes in the strength of the magnetic field of the electromagnet (which occur when switching it on and off) produces a current in the wire connected to coil B (which, remember, is not in electric contact with A). The proof that a current was running through B was the movement of the magnetic needle; it was known from Oersted's work that a current flowing in a wire will move a nearby magnetic needle.
Within a few weeks he further investigated this effect by setting up a cylindrical coil made with 70 meters of wire, and arranging to rapidly move a permanent cylindrical magnet of about 22 centimeters length up and down inside the coil. He then was able to observe an electric current in the coil, induced by the moving magnet. The direction of the current produced in the coil when the magnet was moved into the coil was the reverse of that produced when it was then pulled out. This important experiment proved that moving a wire through a magnetic field produced a current in the wire (because it was logically equivalent to holding the magnet still, and moving the coil instead).
He later succeeding in generating a current by rotating a copper disk between the poles of a large horseshoe magnet; the disk had one wire fixed to its center, and another made sliding contact along the edge of the disk. This was the first generator, and all generators today are its descendants.
Faraday called this effect electromagnetic induction, and he was fascinated by the symmetry revealed by the effect that he now had discovered. Previously, it had been known that a moving electric charge (i.e. a current) produced a magnetic field; now it had been shown that a moving magnet produced an electric field (it is this field which causes the current to flow).
Magnetic induction is of extreme importance in modern industrial society, because it is the principle behind electric generators and transformers.
Chemistry
The basic setup of electrochemistry is simple: it consists of a vessel containing an electrolyte, which is a solution of charged particles (ions). Since this solution is neutral in total, the total charge carried by the positive particles (cations) is he same (in absolute value) as the total charge carried by the negative particles (anions). Two electrodes are dipped into the electrolyte. Outside the vessel a direct current (DC) runs from the negatively charged electrode (the cathode) to the positively charged electrode (the anode). Inside the vessel cations move to the cathode, pick up electrons—which carry negative charge—so that the cations are neutralized and are deposited on the cathode (or if the neutral species is gaseous, it escapes from the cathode in the form of gas). At the anode the opposite happens: anions lose their excess electrons and are deposited. Outside the vessel the electrons run from the anode to the cathode (by definition they run in direction opposite to the DC). Faraday's first law states that the amount of substance deposited on the electrodes is proportional to the amount of current that passed through the electrodes. In the case of a steady current this amount is equal to the time that the current has been running multiplied by the strength of the current. A constant proportional to this amount is called after Faraday—Faraday's constant. Faraday's second law of electrochemistry is a recognition of the fact that anions and cations may carry one, two, three, etc. elementary charges, so that it takes one, two, three, etc., electrons, respectively, to neutralize the cation, while the anion must lose one, two, three, etc. electron to become neutral. These differently charged ions will all give the deposition of one atom only, so that, e.g., twice as much electricity is needed to generate an atom from a doubly charged ion as from a singly charged ion. Faraday was the first to see these facts clearly, moreover, he introduced the corresponding terminology (electrolyte, electrode, anode, cathode, ion, anion, and cation) derived from old Greek, helped by his friend William Whewell of Trinity College, Cambridge, who knew Greek.
Dielectric and Faraday's cage
In his work on electrolysis Faraday noticed that many liquids that conduct an electric current become non-conducting when frozen. For instance (slightly acidic) water is a good conductor, but ice is an insulator. Two oppositely charged plate electrodes attract each other, even if a non-conducting substance, called by Faraday a dielectric, is in between the plates. For Faraday this meant that the electrostatic attraction is not an action at a distance—as was generally assumed at the time—but a force conveyed by contiguous particles. In the second half of the 1830s he started a research program to prove these ideas about lines of forces that are carried by intermediate particles (later called ether), trying to disprove instantaneous action at a distance. The collection of all force lines forms a field of force, a term coined by William Thomson, who advocated and extended Faraday's ideas. To prove his point Faraday constructed capacitors of different shapes and sizes and experimented with all kinds of dielectrics. To honor this work the SI unit of capacitance (the amount of charge that can be stored in a capacator) is called a farad.
In the course of this research program, in November 1837, Faraday had a wooden cube of 4×4×4 feet built and had the sides completely covered with a network of conducting wires. He gives the following description:[13]
I went into the cube and lived in it, and using lighted candles, electrometers, and all other tests of electrical states, I could not find the least influence upon them, or indication of anything particular given by them, though all the time the outside of the cube was powerfully charged, and large sparks and brushes were darting off from every part of its outer surface.
The results of his experiments in the cube enabled him show that electricity was a force rather than an imponderable fluid, as was argued by some physicists at that time. Now we would call the cube a cage of Faraday, notorious among users of cellular phones, saver of car drivers in thunderstorms.
Further reading
- Cantor, Geoffrey N. Michael Faraday: Sandemanian and Scientist Macmillan (1991) - Explores the potential connections between Faraday's religious beliefs and his science.
- Cantor, G. N., David Gooding, and Frank A. J. L. James Michael Faraday (1996)
- Williams, L. Pearce Michael Faraday, A Biography Basic Books, New York (1965) - The first modern scholarly biography.
Notes
- ↑ Simmons, John G. The Scientific 100: A Ranking of the Most Influential Scientists, Past and Present
- ↑ [1] Press Release, University of Bath, 25 October 2006
- ↑ Simmons, John G. The Scientific 100: A Ranking of the Most Influential Scientists, Past and Present, pp. 60, 62 for gasses and electrochemistry, respectively.
- ↑ J.H. Gladstone, Michael Faraday, (3rd ed, 1874). online edition
- ↑ According to Discovery as Invention: Michael Faraday, this was theorized before by others, but he was the first to actually demonstrate it.
- ↑ Frank A. J. L. James, "Faraday, Michael (1791–1867)", Oxford Dictionary of National Biography, Sept 2004; online edn, Jan 2008
- ↑ Frank A. J. L. James, "Faraday, Michael (1791–1867)", Oxford Dictionary of National Biography, Sept 2004; online edn, Jan 2008
- ↑ Farndon, John et al. The Great Scientists: From Euclid to Stephen Hawking, Metro Books, New York (2007) pp. 82
- ↑ M. Faraday, The Chemical History Of A Candle (1908) online edition
- ↑ M. Faraday, On the Various Forces in Nature and their relations to each other: a course of lectures delivered before a juvenile audience at the Royal Institution, (six lectures)online
- ↑ A modification of Plate IV in: M. Faraday, Experimental researches in electricity, vol II, Richard and John Edward Taylor, London (1844). On line
- ↑ Bence Jones, The Life and Letters of Faraday vol. II, online
- ↑ M. Faraday, Experimental researches in electricity, vol I, 2nd edition, Richard and John Edward Taylor, London (1849). p. 366 On line
External links
- Faraday biography in the MacTutor History of Mathematics Archive at the School of Mathematical and Computational Sciences at the University of St Andrews
- Michael Faraday biography at the Institute of Chemistry at the Hebrew University of Jerusalem
- Biography of Faraday (a fairly extensive biography by Dr. Frank A. J. L. James)
- History of the Royal Institution of Great Britain (by Dr. Frank A.J.L. James; has much information on Faraday)