User:Anthony.Sebastian/Life/draft/Sebastian Notes
test
- To do justice to the number of possible different protein structures in a living cell, one would essentially have to discuss the mechanisms involved in producing them according to the Figure 1 in:
- Norregaard Jensen O. (2004) Modification-specific proteomics: characterization of post-translational modifications by mass spectrometry. Current Opinion in Chemical Biology 8:33-41
- Shouldn't we leave such any molecular biology discussion for the appropriate article? Seems that level of technically not needed for the Life article.
- Suggest we change:
“All cells inherit digitally stored information in the form of molecules of DNA, and with minor exceptions the DNA of all uses the same universal genetic code to guide production of thousands of different protein types, manufactured by tiny organelles called ribosomes. Cells use those various proteins to carry out diverse activities, including energy processing and conversion of carbon, nitrogen and phosphorous-containing materials into cellular structures. In the human genome, perhaps as few as 22,000 different protein-coding genes[1] guide the production of the variety and quantity of protein molecules sufficient to scaffold the structures and functions of a cell.”
- to:
"All cells inherit digitally stored information in the form of molecules of DNA, and with minor exceptions the DNA of all use the same universal genetic code to guide production of myriad distinct protein structures, manufactured by tiny organelles called ribosomes. Cells use those proteins to carry out diverse activities, including energy processing and conversion of carbon, nitrogen and phosphorous-containing materials into cellular structures. In the human genome, perhaps as few as 22,000 different protein-coding genes[2] lead to the production of perhaps an order of magnitude greater number of distinct protein structures that make up the variety and quantity of protein molecules needed to scaffold the structures and functions of a cell. Numerous molecular mechanisms account for that quantitative gene-to-protein amplification. [3]
Synthesis of perspectives
The activity of living performed by a cell-based molecular system depends fundamentally on the living system’s maintenance of a near steady-state of organized functioning far from the equilibrium state of randomness. The system can achieve that state in part because of its location in the path of a downhill gradient of flowing energy and/or mass-energy. It exploits that gradient through its abilities both to import a portion of the available energy and/or material flowing past it, and to export unusable energy and material, achieving in the process the outcome of increasing its own level of order at the expense of a more than counterbalancing level of dis-order of its surroundings. It thereby constrains the dissipation of the external energy gradient by exporting a degraded form of energy, or entropy.
Those principles apply to living systems as single cells, as multicellular organs and organisms, and as multi-organism demes and ecosystems.
The building block and working unit of all living systems is the cell. A system’s achievement of order depends on its working unit’s possession as a starting resource informational content — embedded in a macromolecular structure — enabling it to produce a particular suite of molecular components that can respond to the imported external energy and material by organizing itself, solely through physicochemical molecular interactions, for production and maintenance of its far from equilibrium state. That physicochemically-derived far from equilibrium state comprises modular networks of molecular interactions, and networks of interacting networks.
The properties of the networks and the dynamical characteristics of the hierarchy of networks enable the system to act as an autonomous agent, working in its own behalf to perpetuate itself. The self-perpetuation occurs in real-time, inasmuch as the process of natural selection designed molecular networks with properties endowing them with the ability to maintain their steady-state in response to fluctuations in environmental factors. Natural selection, and other evolutionary processes, also designed the networks to regulate the flow of information through the cell, which, among other things, contributes to that real-time self-perpetuation.
Those principles, too, apply to all living systems. An organism, plant or animal, comprises a network of organs working autonomously in its own behalf, maintaining its steady-state functioning far from equilibrium in response to environmental perturbations — a process physiologists refer to as homeostasis.
The collaboration of natural selection and physicochemical interplay serves to perpetuate living systems not only in real-time but also in geological, or ‘evolutionary’, time, as living systems reproduce themselves as systems in unidirectional time. From one universal common ancestor cell — however that may have emerged from non-living systems — informationally-guided, self-organizing, autonomous network dynamics enabled generation of the diversity of all extant living systems on the planet over a period of more than three billion years. Living systems perpetuate living systems, exploiting free energy on its inexorable path to dissipation, constraining that dissipation in developing systems organization by a more than counterbalancing dis-organizing of the larger system in which it is embedded and remains a part.
crescendo
THERMODYNAMIC
A living system possesses the ability to remain for a time in a near steady-state as an organized system, enabled by influx of external energy and matter and by more than compensatory efflux of waste (disorder), thereby exploiting its organizationally enabling far-from-equilibrium state. |
EVOLUTIONARY
A living system possesses the ability to remain for a time in a near steady-state as an organized system, enabled by influx of external energy and matter and by more than compensatory efflux of waste (disorder), thereby exploiting its organizationally enabling far-from-equilibrium state — and capable of participating in the transgenerational evolution of the species to which it belongs in adapting to changing environments. |
SELF-ORGANIZATION
A living system possesses the ability to remain for a time in a near steady-state as a self-organized system, enabled by influx of external energy and matter and by more than compensatory efflux of waste (disorder), thereby exploiting its organizationally enabling far-from-equilibrium state — and capable of participating in the transgenerational evolution of the species to which it belongs in adapting to changing environments. |
AUTONOMOUS AGENTS
A living system possesses the ability to remain for a time in a near steady-state as a self-organized system, working autonomously in its own behalf to offset responses to perturbations, and to reproduce itself, enabled by influx of external energy and matter and by more than compensatory efflux of waste (disorder), thereby exploiting its organizationally enabling far-from-equilibrium state — and capable of participating in the transgenerational evolution of the species to which it belongs in adapting to changing environments. |
NETWORKS
A living system possesses the ability to remain for a time in a near steady-state as a self-organized system of hierarchical robust modular networks, autonomously in its own behalf to offset responses to perturbations, and to reproduce itself, enabled by influx of external energy and matter and by more than compensatory efflux of waste (disorder), thereby exploiting its organizationally enabling far-from-equilibrium state — and capable of participating in the transgenerational evolution of the species to which it belongs in adapting to changing environments. |
INFORMATION
A living system possesses the informational content and information-processing faculty to remain for a time in a near steady-state as a self-organized system of hierarchical robust modular networks, working autonomously in its own behalf to offset responses to perturbations, and to reproduce itself, enabled by influx of external energy and matter and by more than compensatory efflux of waste (disorder), thereby exploiting its organizationally enabling far-from-equilibrium state — and capable of participating in the transgenerational evolution of the species to which it belongs in adapting to changing environments. |
box
Anthony, There are many other parameters too but these few are a good place to start. Chris Day (Talk) 14:41, 26 March 2007 (CDT) |
my text more text |
TEST FIGURE
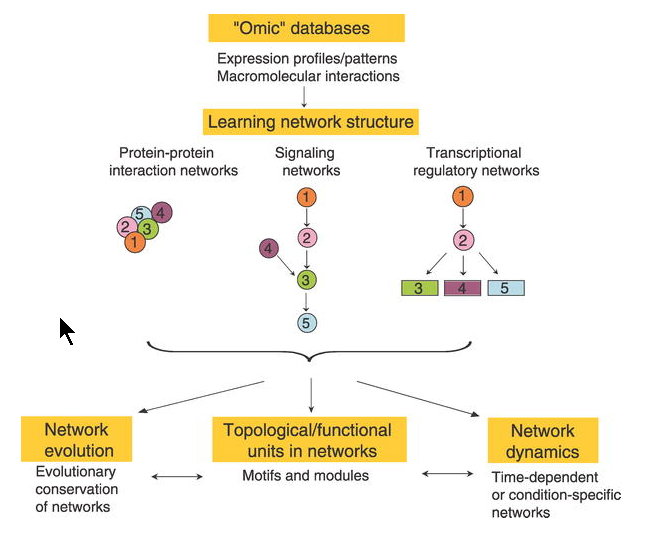
GLOSSARY
- vital force: A discredited hypothesized immaterial force permeating entities that gives them the quality of 'living'. Also referred to as elan vital.
reification semantic primes Archaea Bacteria Eukarya plasma membrane lipid protein energy chemical energy chemical bonds DNA code polypeptide replicate network dynamics coordinated hierarchical gene expression reproduction systems biology biology emergence property function behavior reductionism paradigm organization downward causation reciprocal causation bottom-up top-down thermodynamics heat work entropy information order electromagnetic energy steam engine exhaust random gradient non-equilibrium thermodynamics far-from-equilibrium equilibrium mutation progeny conspecific evolution natural selection descent with modification heritable genotype phenotype RNA meme artificial life genetic programming exobiologist astrobiologist extraterrestrial gene transcription translation molecule covalent hydrogen bonding van der Waals forces supramolecular adaptation metaphorically genome simulation zygote complexity autonomous agent enzyme auto-catalysis allosteric molecular autonomous agents artificial intelligence thermodynamic work cycle node edge arrow link ecosystem gene regulatory circuits modularity robustness motifs exapt autoregulatory circuits feedforward reproductive fitness information pheromones symbols cultural evolution culturgens
The Information Processing Perspective
- Define information
- Relate to entropy
- Define entropy
Self-Organization
Living systems organize themselves spontaneously. Molecular self-organization in cells emerges in part from the chemical properties of the proteins encoded in genes.[4] Those proteins make their appearance by a genetic transcription-translation machinery, which itself represents a self-organized function emerging in part from the chemical properties of proteins and other molecules. Molecules can interact through the formation and breaking of strong covalent bonds and also through weaker, nonstable noncovalent electromagnetic forces, like hydrogen bonding and van der Waals forces and many othersThose weaker ‘supramolecular’ interactions give physical properties to molecular aggregates and make possible many intracellular and intercellular biological processes.[4] [5][6]
The qualifier, ‘in part’, in the preceding paragraph reflects the need to invoke not only molecular self-assembly but also evolutionary mechanisms selecting genes that yield proteins whose chemical properties entail interactions that tend to optimize functional self-organization—in other words, adaption to circumstances. One must also invoke local real-time selective processes that confer stability and appropriate functionality to molecular self-assembly. Self-organization and adaptation functionally conjoin.[7]
One way to the self-organization of cell structure and function is to view the genome metaphorically as a computer, the genome functioning as a ‘program’ that constructs critical components of the cell that can arrange themselves in a way that accords with their chemical properties. With the tinkering comprising local trial-and-error and evolution’s handiwork, that arrangement can then carry out integrative functions not explicitly encoded in the genome itself.[8]
As Oxford professor Denis Noble reminds us, the Nobel Prize-winning geneticist, Sidney Brenner,[9] expressed the computer metaphor this way:
- ”…biological systems can be viewed as special computing devices. This view emerges from considerations of how information is stored in and retrieved from the genes. Genes can only specify the properties of the proteins they code for, and any integrative properties of the system must be 'computed' by their interactions. This provides a framework for analysis by simulation and sets practical bounds on what can be achieved by reductionist models.”[10]
The patterns of structure and behavior that develop in self-organized systems require no master and no prepared recipes that explicitly specify the organized structure and dynamics of the system, which emerge from the interactions among the components dictated by their physical properties as dynamically modified by consequences of the emerging organization embedded in its environment.
Self-organization tends to breed greater degrees of self-organization—breeding more complexity. Genes not only express proteins that organize themselves into a functional unit but also express proteins that organize themselves to regulate that functional unit, as in transcription regulatory circuits. Protein networks interact in a self-organizing way to produce networks of networks with complex levels of coordination. Cells communicate with other cells, either in free-living cellular communities or in multicellular organisms—communicative activities that self-organize in virtue of the properties, functions and behaviors of the cells, selected for fitness by evolutionary mechanisms, and responsive to downward regulation by environmental influences on the whole system.
The single-celled zygote self-organizes into a multicellular living system as the properties of the genetically encoded proteins interact in accord with their physical properties, responding to changing influences stemming from the changing environment generated by growing multicellularity—self-organizing into a network of multiple cell-types doing cooperative work.
Thermodynamic
Biologists sometimes view living things from the perspective of thermodynamics[11]---the science of interactions among energy (capacity to do work, a driving force), heat (thermal energy), work (movement through force), entropy (degree of disorder) and information (degree of order).[12] The interactions define what the system can and cannot do in the process of interconverting energy and work. For example, by the First Law of thermodynamics we know that when a process converts one form of energy to another, it results in no net loss of energy, and no net gain.[13]
Appreciation of the penetrating insight the thermodynamic perspective gives to explaining what constitutes a living entity requires a basic understanding of the laws of thermodynamics that scientists discovered through experiment, debate, mathematical formulation and refinement, and that Albert Einstein believed stood as an edifice of physical theory that could never topple.
Most pertinent, the Second Law of Thermodynamics, has several expressions:
- Heat flows spontaneously—i.e., without external help—from a region or body of higher temperature to one of lower temperature, and never so flows spontaneously in the reverse direction. That holds for other forms of energy as well (e.g., electromagnetic, chemical, etc.)—concentrations of energy disperse themselves to lower energy levels, flowing “into the cool”,[14] so to speak.
- When heat as input to a system causes the system to perform work (e.g., a steam engine), part of the heat disperses as ‘exhaust’, unused and unusable by the system for further work. That holds as well for other forms of energy doing work. Some of the energy always turns into ‘exhaust’, typically heat. Energy conversion to work in a system can never proceed at 100% efficiency—an empirical fact.
- The degree of order or organization of a system and its surroundings cannot spontaneously increase; it either remains the same or decreases. Scientists have learned to put a number on the degree of ΄΄disorder΄΄ of a system, and refer to it as ΄΄entropy΄΄. Water vapor, with its water molecules in more or less random distribution, has a higher entropy value than liquid water, with its molecules distributed less randomly, and has a higher entropy value than ice, with its molecules distributed in a more organized crystal array. Left to itself, ice tends to spontaneously melt, and liquid water to evaporate. Order tends to disorder, with the Universe as a whole tending to exhaust itself into an ‘equilibrium’ state of randomness.
Those three expressions of the Second Law in effect restate each other as tenets of classical thermodynamics reflecting the empirical fact that high energy and order spontaneously flow downhill—down a ‘gradient’—toward eliminating the gradient, as if nature abhors gradients of energy and order.[14] Upon gradient elimination, all energy and order has degraded, all tendencies to change disappear, no further change occurs, an equilibrium state ensues.
Given the universality of the Second Law, how then do living entities manage to come into existence, develop from an ‘embryonic’ state to one of greater degrees of order and lesser degrees of entropy, and to perpetuate their order and increase in order, if only by manufacturing replacements of themselves? How do they thwart the Second Law?
They don’t. They appear to do it by exploiting the Universe’s gradients of energy and order, by their location along those gradients, between the energy input and exhaust. Like a steam engine, they ‘import’ the available energy and order, converting it to the work of internal organization, reducing their entropy. But all along emitting enough exhaust to more than compensatorily increasing the disorder and entropy of their surroundings, so that the total entropy of the living system and its surroundings increase—in keeping with the Second Law.
Science has formalized that concept in the special field of 'non-equilibrium' thermodynamics. It describes many of the characteristics of living systems that remain, for a more or less long time (a lifespan), in a steady-state of organized functional activity. A living system performs its organized functional activities far from the 'equilibrium' state of activity of the system that would obtain if it did not have access to and ability to store and utilize available energy (including mass-energy) from outside the system. Such systems can store energy and perform work on themselves and outside; the available outside energy ultimately supplies the driving force that keeps the system functioning far-from-equilibrium and in disequilibrium with its environment.
Biological cells qualify as non-equilibrium thermodynamic systems because they must consume energy to live, and because they reach an equilibrium state only in death---whereupon all parts relate to each other according to spontaneous physicochemical processes. Viewing living systems from this perspective gives biologists mathematical tools to work with to learn how the system organizes itself. Those tools may help find ways to eliminate accumulated dysfunctions in the organizational activity of a living system that eventually causes it fail to maintain its optimal organization sufficiently to keep the system organized and therefore far-from-equilibrium. Slowing or eliminating dysfunctions of organizational activities might increase lifespan.
We can, then, view a living system as a state of organizational activity (non-randomness) that is maintained by importing, storing and transforming energy and matter from its external environment into the work and structures required to sustain its organizational activity. In doing so, living systems produce waste and export it to the external environment, lowering the organizational state of the environment. The living system thus maintains its internal organization at the expense of that of the external environment, leaving the environment more disorganized than the gain in organization of the living system--in keeping with the second law of thermodynamics that the total disorder (system plus environment) always increases.
Thus, the following could serves as a fundamental characterization of life, or of living systems:
- The ability to remain for a time (a "lifespan") as an organized, coordinated functioning system, in which spontaneous tendencies and external forces that tend to disturb its organization are opposed by built-in self-correcting mechanisms fueled by external resources (energy, matter) and facilitated by production and exportation of waste (disorder)---thus all the while operating far-from an ever-approaching equilibrium (the state that we call "death").
Test Box
my text more text |
darwin
Last Paragraph Charles Darwin’s Origin of Species (1st edition, 1859) It is interesting to contemplate an entangled bank, clothed with many plants of many kinds, with birds singing on the bushes, with various insects flitting about, and with worms crawling through the damp earth, and to reflect that these elaborately constructed forms, so different from each other, and dependent on each other in so complex a manner, have all been produced by laws acting around us. These laws, taken in the largest sense, being Growth with Reproduction; Inheritance which is almost implied by reproduction; Variability from the indirect and direct action of the external conditions of life, and from use and disuse; a Ratio of Increase so high as to lead to a Struggle for Life, and as a consequence to Natural Selection, entailing Divergence of Character and the Extinction of less-improved forms. Thus, from the war of nature, from famine and death, the most exalted object which we are capable of conceiving, namely, the production of the higher animals, directly follows. There is grandeur in this view of life, with its several powers, having been originally breathed into a few forms or into one; and that, whilst this planet has gone cycling on according to the fixed law of gravity, from so simple a beginning endless forms most beautiful and most wonderful have been, and are being, evolved. |
- text
- text
text text text
new
Biologists use the term life to refer to the processes comprising the activity of living, to the entities that embody those processes, and to the interrelations and interactions among them, that together, form complex adaptive self-reproducing systems. The question “what is life?” then turns on just what, precisely, characterizes those 'processes of living'. In answering that, biologists hope to find answers to many other questions in biology, including perhaps, some not yet even asked (see Biology and Systems biology). The meaning of the terms 'life' and 'living' in a number of different contexts unfolds in the course of the article.

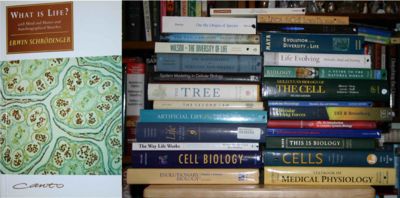
....
The words 'life' and 'living' require clear thinking
Ernst Mayr, a 20th century giant among evolutionary biologists, in his last decade as a centenarian, wrote a book called This is Biology: The Science of the Living World.[15] In the opening chapter, What Is the Meaning of “Life” , he declares that understanding 'life' is one of the major objectives of biology. However, he suggests that we should fuss less about defining 'life', and concentrate more on defining the process of 'living':
"The problem here is that 'life' suggests some 'thing' — a substance or force — and for centuries philosophers and biologists have tried to identify this 'vital force', to no avail. In reality, the noun 'life' is merely a reification of the process of living. It does not exist as an independent entity. One can deal with the process of living scientifically, something one cannot do with the abstraction 'life'. One can describe, and even try to define, what living is and what a living organism is, and one can try to make a demarcation between living and nonliving. Indeed, one can even try to explain how living as a process can be the product of molecules that themselves are not living."
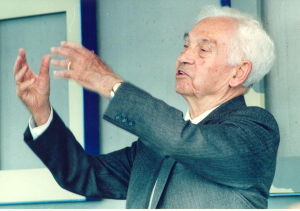
Scientist Eric Schneider and science writer Dorian Sagan echo Mayr:
"...the word is a grammatical misnomer: life is a noun, but the phenomenon to which it refers is a process. And it is vitalistic: when we say life, we think we know what we are talking about when often we have simply applied a label that allows us to categorize, rather than examine closely, the phenomenon about which we are speaking."[14]
Why this concern about the word? Some words have distinct meanings not definable in terms of other words, and those essential words semioticians call ‘semantic primes’. Ultimately, all definitions converge on about 70 so-called 'semantic primes', universal among languages. Children learn the meaning of prime words by how the society in which they live use them in every day language. Every other word can be defined using some combination of semantic primes.[16] The verb ‘live’ is a semantic prime, the noun ‘life’ is not. [17]
Using semantic primes, 'Life' is defined as 'that which lives', where lives is understood by speakers and listeners from their experience with the language-speakers in their environment. The word "death" also comes from the semantic prime of 'that which lives'. Things which live 'die' and speakers generate the word 'death' to refer to 'that which died'.
Semantically, the question, then, is not 'what is life?', but 'what characterizes things that live?' Biologists act on the latter question, even as they ask it in terms of the former. The biologist, logician and historian J.H. Woodger suggested that the word 'life' can be eliminated from the scientific vocabulary, because it is "an indefinable abstraction and we can get along perfectly well with 'living organism', which is an entity that can be speculatively demonstrated.”[18] Carol Cleland, philosopher and member of NASA’s Astrobiology Institute, adds that scientists are not really interested in what the word 'life' happens to mean in our language. "What we really need to focus on is coming up with an adequately general theory of living systems, as opposed to a definition of 'life'."[19] Perhaps this resonates with the remark of biologist Antonio Lazcano: "Life is like music; you can describe it but not define it".[20]
References
Citations and Notes
- ↑ How Many Genes Are in the Human Genome? at the Human Genome Project Information website hosted by the U.S. Department of Energy
- ↑ How Many Genes Are in the Human Genome? at the Human Genome Project Information website hosted by the U.S. Department of Energy
- ↑ (a) The UniProtKB/Swiss-Prot Human Proteome Initiative [1]; (b) Norregaard Jensen O. (2004) Modification-specific proteomics: characterization of post-translational modifications by mass spectrometry. Current Opinion in Chemical Biology 8:33-41
- ↑ 4.0 4.1 Lehn JM. (2002) Toward Self-Organization and Complex Matter. Science 295:2400-3. Link to Full-Text
- ↑ Reinhoudt DN, Crego-Calama M. (2002) Synthesis Beyond the Molecule. Science 295:2403-7 Link to Article
- ↑ Percec V, Ungar G, Peterca M. (2006) CHEMISTRY: Self-Assembly in Action. Science 313:55-6 Link to Article
- ↑ Heylighen F. (2001) The Science of Self-organization and Adaptivity. In: Kiel LD, ed. Knowledge Management, Organizational Intelligence and Learning, and Complexity: The Encyclopedia of Life Support Systems ((EOLSS). Oxford: Eolss Link to Full-Text
- ↑ Noble D. (2002) Modeling the Heart--from Genes to Cells to the Whole Organ. Science 295:1678-82 Link to Full-Text
- ↑ Link to full-text of Sidney Brenner’s Nobel lecture “Nature’s Gift to Science” 2002
- ↑ Brenner S. (1998) Biological computation. Novartis.Found.Symp. 213:106-11 PMID 9653718
- ↑ Note: thermodynamics: thermo-, heat; -dynamics, movement
- ↑ Note: A random pattern of parts has no order and has no information—it has maximal entropy and zero information. A living system has order in its organized functions, has computationally-rich informational content, and low entropy.
- ↑ Note: The total energy of the Universe remains constant, but if and when it completely disperses itself, it no longer can do work.
- ↑ 14.0 14.1 14.2 Schneider ED, Sagan D. (2005) Into the Cool: Energy Flow, Thermodynamics, and Life. Chicago: The University of Chicago Press. ISBN 0-226-73937-6 Link to Chapter Excerpts and Reviews Cite error: Invalid
<ref>
tag; name "schneider05" defined multiple times with different content - ↑ Mayr E (1997) This is Biology: The Science of the Living World. Cambridge, Mass: Belknap Press of Harvard University Press
- ↑ Wierzbicka A (1996) Semantics: Primes and Universals. Oxford England: Oxford University Press ISBN 0198700024
- ↑ See list of semantic primes at this site: Goddard C, Wierzbicka A (2006) Semantic Primes and Cultural Scripts in Language: Learning and Intercultural Communication
- ↑ J.H. Woodger (1929) quoted in Barbieri M (2003) The Organic Codes; An Introduction to Semantic Biology. Cambridge: Cambridge University Press. Appendix. DEFINITIONS OF LIFE. (Author notes: From Noam Lahav's Biogenesis, 1999; from Martino Rizzotti's Defining Life, 1996; and from personal communications by David Abel, Pietro Ramellini and Edward Trifonov, with permission)
- ↑ Read Carol Cleland here
- ↑ (Lazcano 1994, cited in Popa R (2004) Chronology of Definitions and Interpretations of Life. In: Popa R, ed. Between Necessity and Probability: Searching for the Definition and Origin of Life. Berlin: Springer-Verlag 2004: pp 197-205